Why did Sauropods grow so big?
Sauropods are a group of magnificent, long-necked dinosaurs that are well known for their gigantism. Existing from the end of the Triassic to the end of the Cretaceous (201 to 66 million years ago), they hold special places in the hearts and imaginations of dinosaur lovers around the world thanks to their unique body shapes and their ability to achieve such vast numbers on the scales. For example, famous genera such as Diplodocus and Brachiosaurus measured up to 26m in length, and weighed 14,800kg and 46,000kg respectively.
These colossal sizes have puzzled scientists for years: how had creatures this large survived? And to that effect, how had they thrived to such an extent that numerous lineages, groups, and species were able to co-exist? Researchers have extensively studied the fossil remains of these giants, determining diet from their teeth anatomy, or locomotion from trackways.
In 2013, Sander published a review paper exploring an ‘evolutionary cascade model’ comprising five trait cascades, that explain potential factors in sauropod gigantism. An evolutionary cascade model is a method scientists use to consider how changes in one part of an ecosystem can affect other parts of the ecosystem over time. It can be thought of as a chain reaction. In the case of sauropods, a small change such as a new characteristic or trait can trigger other changes that influence survival and behaviour of subsequent lineages.
All of the cascades from Sander, 2013 are linked: former traits provide selective advantages that allow evolution of subsequent traits. This creates a feedback loop, where traits originating higher within the cascade affect lower traits, all eventually leading to very high body mass.
The five evolutionary cascades, are based on:
- Head and neck
- Feeding
- Avian-style respiration
- Metabolism
- Reproduction
The above can be categorised into ancestral traits (reproduction and feeding), and derived traits (avian-style respiration and metabolism) which are all explored in greater detail below.
1 – Head and neck
A characteristic that sauropods are well known for is the development of their distinctively long necks. Not only were they long, but the necks were remarkably flexible, contributing to an increased feeding envelope. This was a key adaptation that contributed to their gigantism.
A feeding envelope is defined by the range over which an organism can successfully access, obtain, and consume food. The greater the feeding envelope of an organism when it is stationary, the less overall energy is used. Already, we can form a hypothesis that perhaps sauropods could remain stationary in one place and only move their necks in order to access food, and thus reduce energy expenditure. But how truly flexible were these necks?
There has actually been a lot of debate over the range of movement of the sauropod neck. Manipulation of cervical vertebrae (neck bones) has revealed that sauropod necks were possibly not as flexible as modern long-necked counterparts. The exact degree of flexibility would, of course, depend on the individual anatomy of each species.
As an example, a study by Cobley et al., 2013 compared the flexibility of sauropod necks to that of modern day ostriches. They argued that calculations of flexibility should take into account soft tissues, and not be based on osteology (the bones) alone as it does not provide an accurate picture. As a result, they concluded that sauropod necks are less flexible than previous studies had thought.
However, a subsequent paper published by Taylor et al., 2014 suggested that the presence of cartilage between the joints allowed sauropod necks to flex beyond 90 degrees, and thus when the soft tissue is added, it revealed that sauropod necks actually were more flexible than previously thought.
The above highlights the importance of considering all factors that contribute to neck flexibility of sauropods. Despite the current inconclusive nature of exactly how flexible the sauropod neck is, we can assume with relative safety that sauropods had a large feeding envelope that reduced energy expenditure. Coupled with their proportionally small heads (that required less energy investment to support as less musculature was needed) Sauropods were able to grow to remarkable sizes.
2- Feeding
We have considered the feeding envelope, but the actual method of sauropod feeding is another entire topic in itself! During their evolution from earlier prosauropods to the famous colossal creatures that are such fan favourites, sauropods gradually lost their ability to chew, reflected in the loss of their adductor muscles.
Countless studies have been done on the morphology of sauropod craniodental features (Calvo, 1994; Christiansen, 2000; Upchurch and Barrett, 2000; Whitlock et al. 2011; Young et al. 2012; Button et al. 2014) much of which go on to analyse the feeding mechanisms of various sauropod species. For example, didplodocids were established to be particularly unusual, utilising a form of branch stripping opposed to the more common methods of cropping vegetation as found in Camarasaurus and Dicraeosaurus (Christiansen, 2000).
But, despite these differences, what all these more derived sauropods had in common was the fact that none of them chewed their food (I know, my grandmother would be appalled!). Alongside the loss of the adductor muscles, sauropod dental morphology adapted to specialise in the stripping and cropping of vegetation, they were not designed for chewing.
There have been proposals of gastroliths, like those found in modern birds. A gastrolith is a small, smooth rounded stone that certain animals will intentionally swallow. These stones then aid digestion through breaking down eaten material through grinding within the stomach. However, there is little evidence of this within the fossil record, and the few examples are thought to be chance encounters.
So what are the benefits of simply ‘inhaling’ your food? This again leads to the idea of lower levels of energy expenditure, but also the need to meet excessively high levels of their basal metabolic rate (BMR – as explored later). It’s thought that these dinosaurs would have needed to eat thousands of kilograms of vegetation a day to meet regular maintenance levels. Abandoning the need to chew was a far more efficient method of eating, and so their bodies became able to handle their ever-increasing size.
3 – Avian Style Respiration
Many dinosaurs do not breathe like we do today, but share the unidirectional (one-way) respiration system of birds. This system of respiration is beneficial for high-energy demands like those experienced by large dinosaurs, such as the sauropods, because it allows the extraction of greater amounts and a constant supply of oxygen. The air sacs, alternatively known as pneumatic foramina when considered in their osteological form as little holes within the skeleton that would have housed the sacs, are also weight saving.
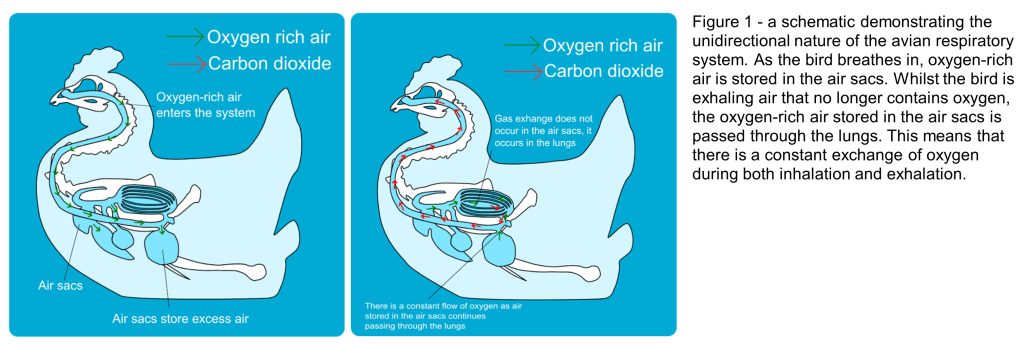
The system works through a two-step process as air only flows in one direction through the respiratory system (Fig. 1). During inhalation, the air travels to the posterior air sacs. As the bird exhales, all the air stored in these posterior sacs is directed to the lungs. Here, the exchange of oxygen and carbon dioxide takes place in the blood within the lung air sacs. The carbon dioxide dominated air is then directed to anterior air sacs so that when the bird inhales again, fresh oxygen can flow into the lungs. In addition to this process, birds also have a system of air sacs that aid movement of air through the respiratory system.
Evidence has been found within fossilised bones of pneumatic foramina (Fig.2). Saltasaurine titanosaurs exhibit extreme cases of this throughout the skeleton (Cerda et al. 2012), demonstrating that larger sauropods did use this method as a form of mass reduction (Broklehurst et al. 2018; Taylor & Wedel, 2021). Additionally, morphological modelling has established that sauropods potentially had a furrowed thoracic ceiling within their lungs (Brocklehust et al. 2018) like birds do that provide a greater surface area, and so more room for gas exchange to take place.
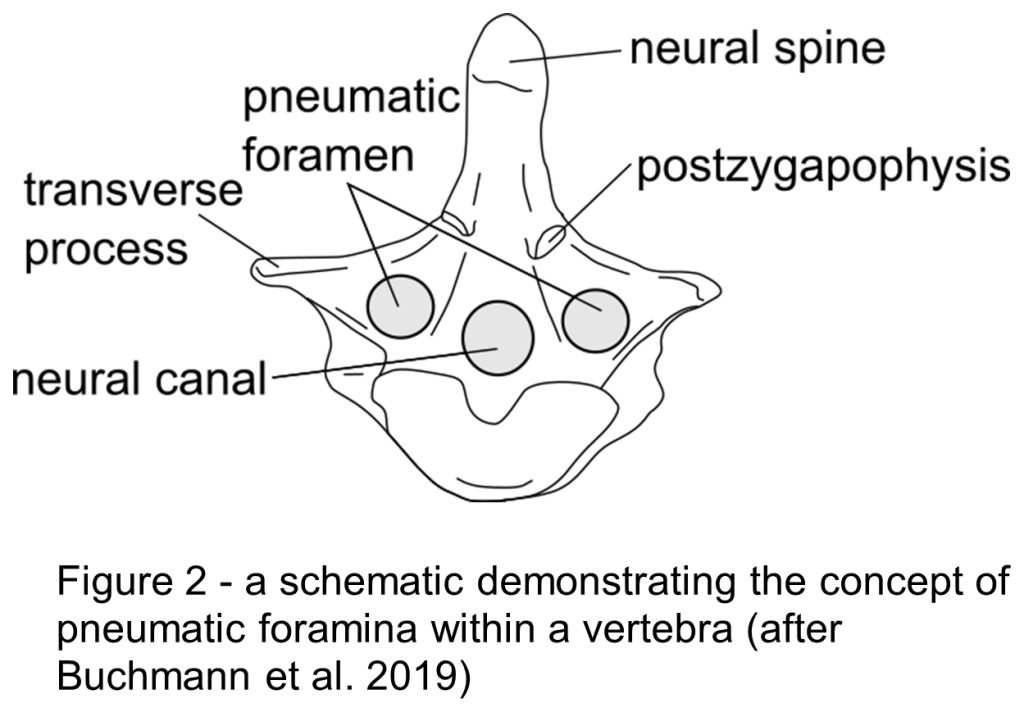
Overall, this style of respiration did contribute to sauropod’s gigantism, but interestingly, it’s thought to be unlikely that the key features of this system were developed entirely for the purpose of growing large. This is because pneumatic foramina have been found within the smallest sauropod clades (Cerda et al. 2012), so why would small sauropods need mass reduction?
There are several proposed reasons for this. The first is that pneumaticityis potentially an ancestral trait from deeper within the archosaur lineage, as it is also a feature found within therapods (O’Conner, 2006). Therefore, it is possible the trait evolved before prosauropods were a fully established group, highlighting that the weight saving advantage was not a primary factor.
Additional factors that have been proposed include the need for improved locomotory control for bipeds, thus allowing a lower centre of mass (Carrier et al. 2001; Farmer, 2006), thermal regulation such as a more efficient method of heat transfer (Sereno et al. 2008), or overall greater efficiency of breathing which smaller prosauropods also would have benefitted from if they also had higher metabolic rates (Ruben et al. 1997; Ruben et al. 1999).
Lastly, some scientists have proposed that these pneumatic structures could have been used for vocalizations, allowing for more complex and louder calls. This could have had social or reproductive benefits (Lockner & Murrish,1975; Dantzker et al. 1999; Plummer & Goller, 2008).
4- High Basal Metabolic Rate (BMR) and rapid growth
The Basal Metabolic Rate (BMR) of sauropod dinosaurs is highly contested and is one of the most poorly constrained out of all dinosaurs. BMR refers to the amount of energy a body requires to perform basic, essential functions whilst at rest, such as thermoregulation (maintaining body temperature) and organ function. Palaeontologists long struggled with the question of what the BMR for sauropods might have been, and how such large creatures managed their body temperature.
Some studies have proposed that a sauropod’s body temperature did not increase with its body mass, instead suggesting a potential inverse relationship (Gillooly et al. 2006; Griebeler, 2013). This would indicate that sauropods must have had an efficient way to cool themselves to prevent overheating, and additionally, the implications of this would also mean that the sauropods require less energy to maintain a high BMR. But what was the efficient cooling system? Some palaeontologists have proposed that the combination of the long neck and previously discussed avian respiration system was a suitable thermoregulation system.
In terms of rapid growth, there have been lots of studies indicating that sauropods did quickly mature, demonstrated by evidence showing them growing much faster than their closest living relatives, and other extinct animals that were not dinosaurs. Rapid growth meant that there was a greater likelihood that juveniles would survive to adulthood and they would quickly acquire the beneficial traits the being so large provided.
5- Large number of eggs and R-selection
In a similar vein to the above, in order to have the greatest chance of passing on their genes, Sauropods laid large numbers of eggs. Perhaps counter-intuitively, these eggs were very small relative to their parents. This was likely because, if the eggs were too large, the shell would be too thick for the juvenile to break through in order to hatch. Unfortunately, small eggs are at the disadvantage of being crushed by inattentive, R-selective parents, opposed to K-selective parents who nurture their young. But, as discussed, juveniles undertook rapid growth so there was a smaller period of time for them to be susceptible to predators and getting stood on.
R-selection and K-selection are an ecological terms. R-selection refers to the highly reproductive parenting strategy, where the offspring are normally small and do not receive much parental care. This method of parenting leads to larger body mass because R-selection methods reduce the energy input required by the parents, and thus overall energy is preserved. Large animals are not limited to these approaches however. Whales and elephants are K-selective, they invest time and energy into caring for their young. This highlights that adopting R-selection is potentially not a significant factor contributing to large body mass.
Conclusion
In conclusion, sauropods were not well adapted to not only maintain their large body size, but to efficiently thrive as one of the most successful lineages of giants to roam the earth. Their gigantism has puzzled scientists for years, leading to a quest for answers about how these immense creatures not only survived but thrived.
The “evolutionary cascade model,” proposed by Sander (2013), provides a compelling framework to understand sauropod gigantism. The model clearly demonstrates that each one of the five developed traits, a long neck and small head, bulk feeding, avian-style respiration, high BMR, and R-selective reproduction, was a scheme in improving efficiency. More efficient methods of browsing, breathing, and reproducing allowed sauropods to evolve to their colossal size.
The sauropods’ long and flexible necks expanded their feeding envelopes, reducing energy expenditure and aiding their gigantism. These herbivores abandoned chewing in favour of more efficient methods of feeding, optimizing energy utilization. Their avian-style respiration system, with pneumatic foramina and a unique air sac network, not only reduced weight but also contributed to their ability to extract sufficient oxygen for their massive bodies.
The debate surrounding sauropods’ Basal Metabolic Rate (BMR) and thermoregulation underscores the complex mechanisms they employed to manage their energy needs. Rapid growth and the production of numerous small eggs optimized their reproductive strategy, increasing the chances of juvenile survival.
While sauropods reached astonishing sizes, questions remain about their eventual extinction, the possibility of even larger sizes, and whether they were approaching the limits of terrestrial gigantism. Future research promises to unravel more mysteries of these remarkable giants that once roamed our planet, leaving an indelible mark on Earth’s evolutionary history.
Bibliography
Primarily based on Sander, 2013.
BROCKLEHURST, R. J., SCHACHNER, E. R. & SELLERS, W. I. 2018. Vertebral morphometrics and lung structure in non-avian dinosaurs. R Soc Open Sci, 5, 180983.
BUCHMANN, R., AVILLA, L. D. S., RODRIGUES, T. 2019. Comparative analysis of the vertebral pneumatization in pterosaurs (Reptilia: Pterosauria) and extant birds (Avialae: Neornithes). PLOS ONE, 14, 10
BUTTON, D. J., RAYFIELD, E. J. & BARRETT, P. M. 2014. Cranial biomechanics underpins high sauropod diversity in resource-poor environments. Proceedings of the Royal Society B: Biological Sciences, 281, 20142114.
CALVO, J. 1994. Jaw mechanics in sauropod dinosaurs. GAIA, 10, 183-193.
CERDA, I. A., SALGADO, L. & POWELL, J. E. 2012. Extreme postcranial pneumaticity in sauropod dinosaurs from South America. Paläontologische Zeitschrift, 86, 441-449.
CHRISTIANSEN, P. 2000. Feeding mechanisms of the sauropod dinosaurs Brachiosaurus, Camarasaurus, Diplodocus and Dicraeosaurus. Historical Biology, 14, 137-152.
COBLEY, M. J., RAYFIELD, E. J. & BARRETT, P. M. 2013. Inter-Vertebral Flexibility of the Ostrich Neck: Implications for Estimating Sauropod Neck Flexibility. PLOS ONE, 8, e72187.
GILLOOLY, J. F., ALLEN, A. P. & CHARNOV, E. L. 2006. Dinosaur Fossils Predict Body Temperatures. PLOS Biology, 4, e248.
GRIEBELER, E. M. 2013. Body temperatures in dinosaurs: what can growth curves tell us? PLoS One, 8, e74317.
O’CONNOR, P. M. 2006. Postcranial pneumaticity: An evaluation of soft-tissue influences on the postcranial skeleton and the reconstruction of pulmonary anatomy in archosaurs. Journal of Morphology, 267, 1199-1226.
O’CONNOR, P. M. 2009. Evolution of archosaurian body plans: skeletal adaptations of an air-sac-based breathing apparatus in birds and other archosaurs. Journal of Experimental Zoology Part A: Ecological Genetics and Physiology, 311A, 629-646.
RUBEN, J. A., DAL SASSO, C., GEIST, N. R., HILLENIUS, W. J., JONES, T. D. & SIGNORE, M. 1999. Pulmonary function and metabolic physiology of theropod dinosaurs. Science, 283, 514-6.
RUBEN, J. A., JONES, T. D., GEIST, N. R. & HILLENIUS, W. J. 1997. Lung Structure and Ventilation in Theropod Dinosaurs and Early Birds. Science, 278, 1267-1270.
SANDER, P. M. 2013. An Evolutionary Cascade Model for Sauropod Dinosaur Gigantism – Overview, Update and Tests. PLOS ONE, 8, e78573.
SERENO, P. C., MARTINEZ, R. N., WILSON, J. A., VARRICCHIO, D. J., ALCOBER, O. A. & LARSSON, H. C. E. 2008. Evidence for Avian Intrathoracic Air Sacs in a New Predatory Dinosaur from Argentina. PLOS ONE, 3, e3303.
TAYLOR, M. & WEDEL, M. Why is vertebral pneumaticity in sauropod dinosaurs so variable? Qeios.
TAYLOR, M. P. 2014. Quantifying the effect of intervertebral cartilage on neutral posture in the necks of sauropod dinosaurs. PeerJ, 2, e712.
UPCHURCH, P. & BARRETT, P. M. 2000. The evolution of sauropod feeding mechanisms. In: SUES, H.-D. (ed.) Evolution of Herbivory in Terrestrial Vertebrates: Perspectives from the Fossil Record. Cambridge: Cambridge University Press.
WHITLOCK, J. A. 2011. Inferences of Diplodocoid (Sauropoda: Dinosauria) Feeding Behavior from Snout Shape and Microwear Analyses. PLOS ONE, 6, e18304.
YOUNG, M. T., RAYFIELD, E. J., HOLLIDAY, C. M., WITMER, L. M., BUTTON, D. J., UPCHURCH, P. & BARRETT, P. M. 2012. Cranial biomechanics of Diplodocus (Dinosauria, Sauropoda): testing hypotheses of feeding behaviour in an extinct megaherbivore. Naturwissenschaften, 99, 637-43.
My name is Harrie Williams, and I am currently a PhD student at the University of Liverpool delving into the fascinating world of mammal jaws, exploring the biomechanics and evolution of both hard and soft tissues. While specialising in this fascinating field, I also hold a deep-rooted interest in Palaeobiology, evolution, and especially fossil hunting! Beyond academia, I am an avid bookworm and enjoy writing my own stories.