Episode 60: Determining Diet
Diet is perhaps the most important aspect of ecology. As such, understanding the diet of extinct animals is crucial if we wish to reconstruct the ecosystems of the past. However, determining what was on the menu for extinct animals, known only from fragmentary fossils, is far from straight forward. We spoke to Dr David Button, from the University of Birmingham, to learn about the techniques palaeontologists use to deduce diet from fossils.
Podcast: Download (Duration: 40:51 — 56.1MB)
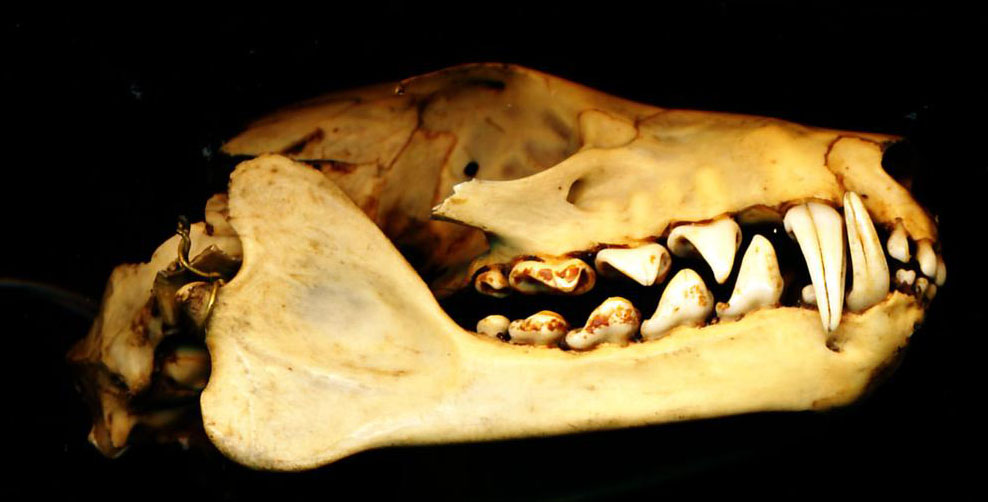
Tooth morphology (the shape of the teeth) can be used to infer wether an organism was a carnivore or herbivore. Carnivores tend to have sharp teeth whilst herbivores usually have flat, peg or leaf-shaped teeth, depending on their diet. Based on the teeth in this skull, we would infer that the owner was a carnivore. Image credit: Naturalis. Image from Wikimedia CC0 1.0.
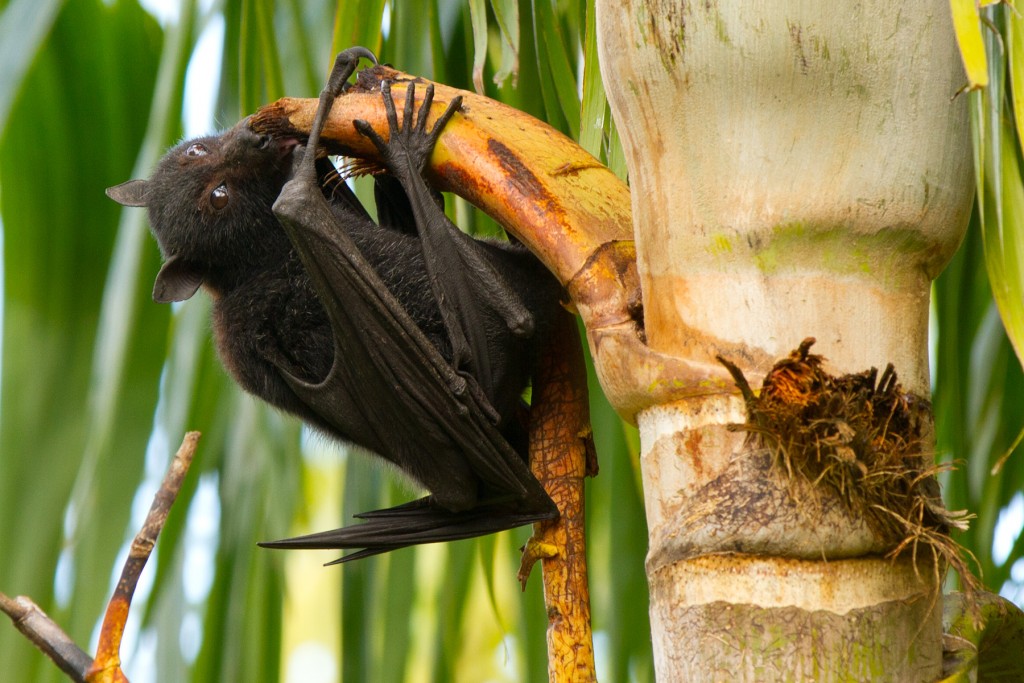
However, tooth morphology does not necessarily correlate with diet. The skull in the above image belongs to a flying fox, which has a diet consisting almost exclusively of fruit (and occasionally palm trees, as seen in this image). Image credit: Andrew Mercer. Image from Wikimedia CC BY-SA 4.0.
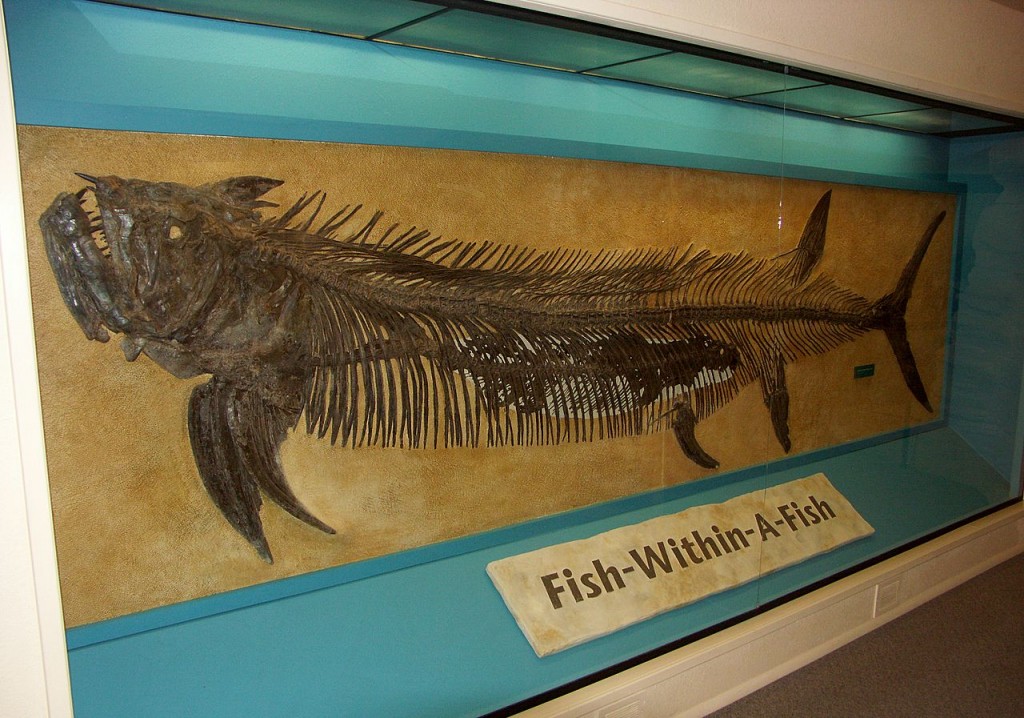
Palaeontologists must consider both direct and indirect evidence in order to infer diet. Direct evidence includes gut contents, where a fossil is preserved with its last meal still inside it. Perhaps the most famous case is the fish-within-a-fish fossil discovered by George F. Sternberg. Here a 4m long Xiphactinus fish is preserved with a 2m long Gillicus arcuatus in its stomach cavity. However, we must be cautious when interpreting these fossils as they may not represent the organisms usual diet. In the case of Xiphactinus, it is suggested that the fish bit of more than it could chew; killed by consuming such large prey. Image credit: Spacini. Image from Wikimedia CC-BY-SA 3.0.
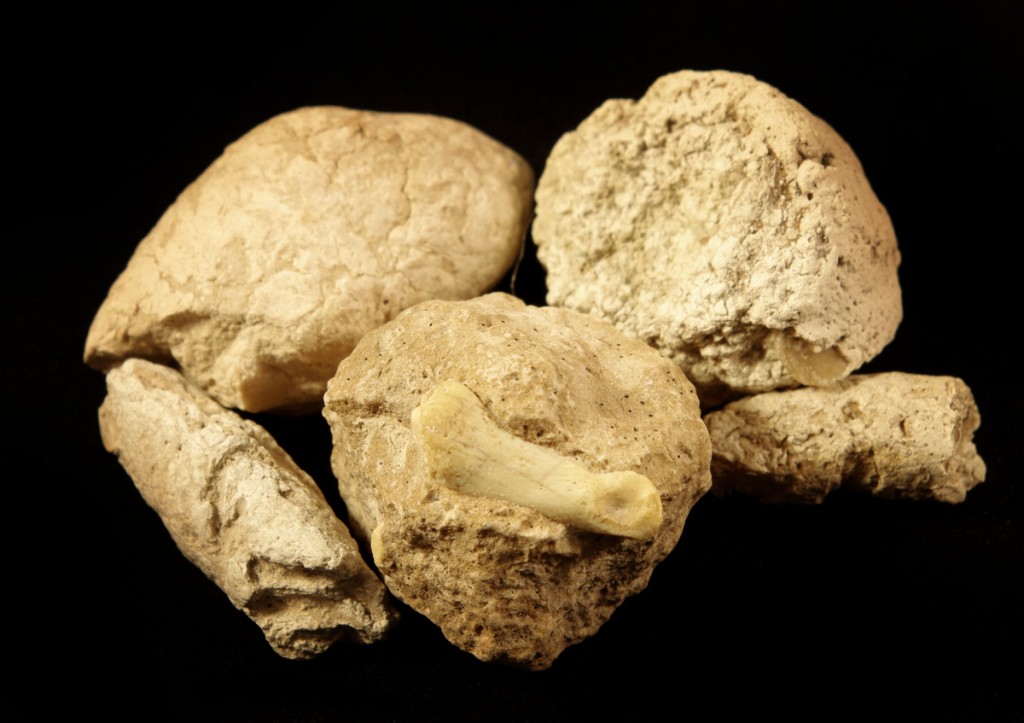
Coprolites (fossil poo) offer another source of direct evidence. This example, from the Oligocene of White River, Nebraska, clearly belongs to a carnivore, as it contains a toe bone of a small deer. However assigning coprolites to a particular species is very difficult. As such, their usefulness in determining the ecology of ancient environments is limited. Image credit: Poozeum. Image from Wikimedia CC BY-SA 4.0.
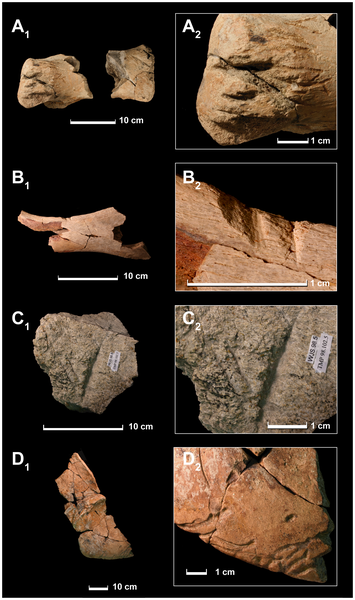
Indirect evidence includes tooth marks on skeletons, which indicate an organism was consumed, either by a predator or scavenger. If these marks are partially healed, it suggests that the animal survived the inflicted wound – identifying the wound maker as a predator. Determining the organism that produced the marks requires close comparison between the shape of teeth, and the shape of the tooth marks. In this image, we can see a variety of scratches and marks associated with T.rex feeding. A, Hadrosaurid metatarsal. B, fragment of hadrosaurid pubis. C, ceratopsid? frill element showing tooth mark. D,Triceratops right squamosal showing tooth marks on edge. Figure from Longrich et al. (2010) Cannibalism in Tyrannosaurus rex. Plos One 5(10) doi:10.1371/journal.pone.0013419.g001
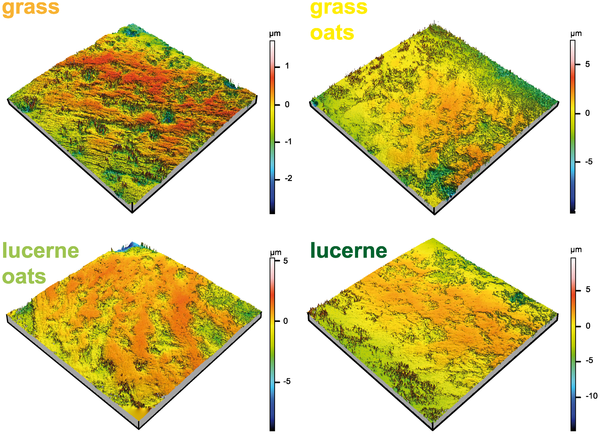
Another source of indirect evidence is large and small-scale wear patterns found on teeth – called macro and microwear respectively. Studies based on living organisms have found that different diets produce markedly different wear patterns. The above figure shows the results of a 25 week long feeding experiment conducted on rabbits. Groups of rabbits were fed exclusively on one of four diets (grass, grass oats, lucerne oats and lucerne). After 25 weeks, the microwear on their teeth were compared. The study revealed that microwear patterns were distinct: grass-fed rabbits showed a high proportion of scratches, while the lucerne-fed group showed a high proportion of pits. Such studies can be used to interpret the wear patterns found on fossil teeth. From Schulz et al. (2013) Dietary Abrasiveness Is Associated with Variability of Microwear and Dental Surface Texture in Rabbits. Plos One 8(2) doi:10.1371/journal.pone.0056167.g003
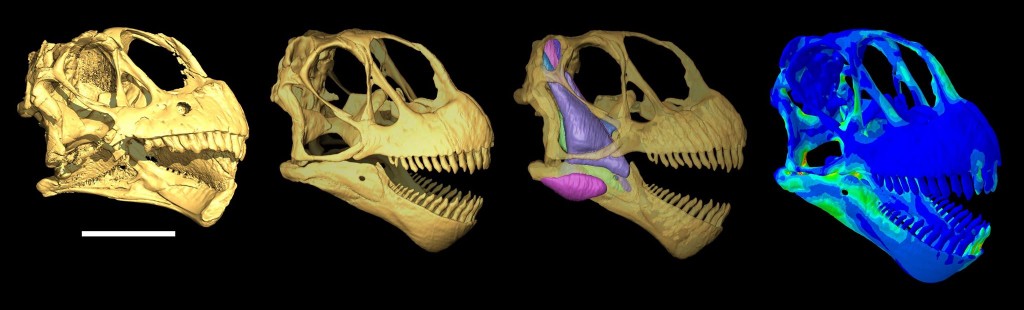
For David’s PhD, he investigated the feeding ecology of sauropod dinosaurs using cranial biomechanics. To do this, David produced computer models of sauropod skulls allowing the mechanical properties of the skulls to be explored virtually. The figure above illustrates the steps involved in biomechanical analysis of the skull of Camarasaurus lentus. Left to right: surface render of the original crushed specimen (CMNH11338); virtual reconstruction of the skull and jaw; virtual reconstruction of the jaw muscles; finite element analysis contour von Mises stress plot. Hotter colours refer to higher stresses. Scale bar = 10cm. Image credit: David Button. Modified from Button, David J., Emily J. Rayfield, and Paul M. Barrett. “Cranial biomechanics underpins high sauropod diversity in resource-poor environments.” Proceedings of the Royal Society of London B: Biological Sciences 281.1795 (2014): 20142114.
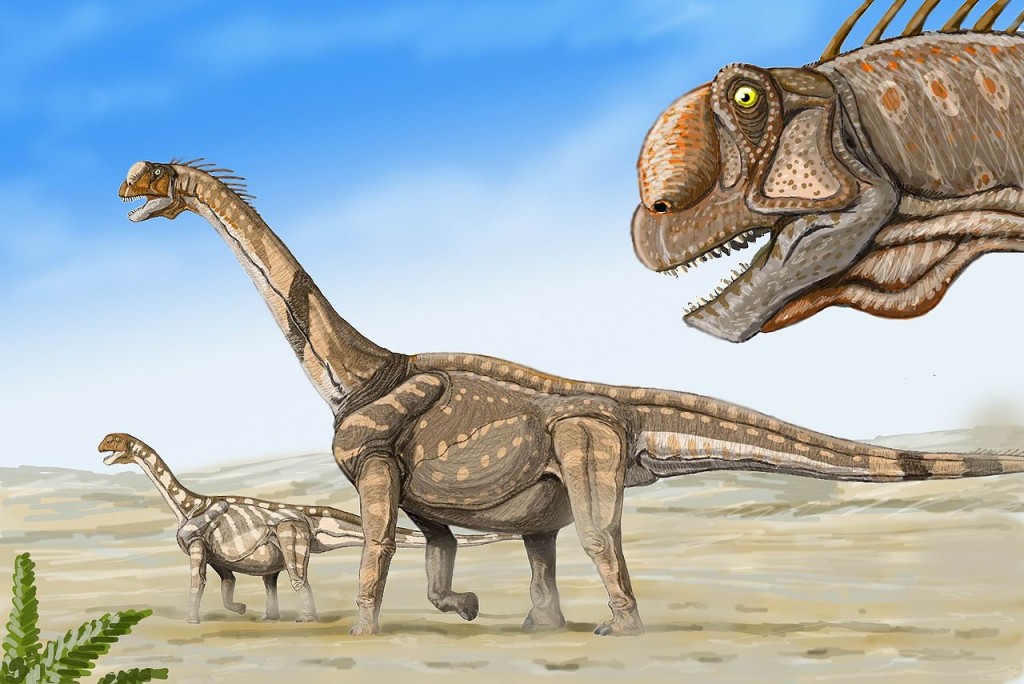
David studied the cranial biomechanics of sauropods from the Upper Jurassic Morrison Formation, in the western United States. This formation is famous for its extremely diverse dinosaur fauna, including a number of large sauropods such as Brachiosaurus, Diplodocus, Supersaurus and Camarasaurus (pictured). Surprisingly, the environment in which these dinosaurs lived was not a lush tropical forest, but instead was semi arid and highly seasonal. Image credit: dmitrchel@mail.ru. Image from Wikimedia CC BY 3.0.
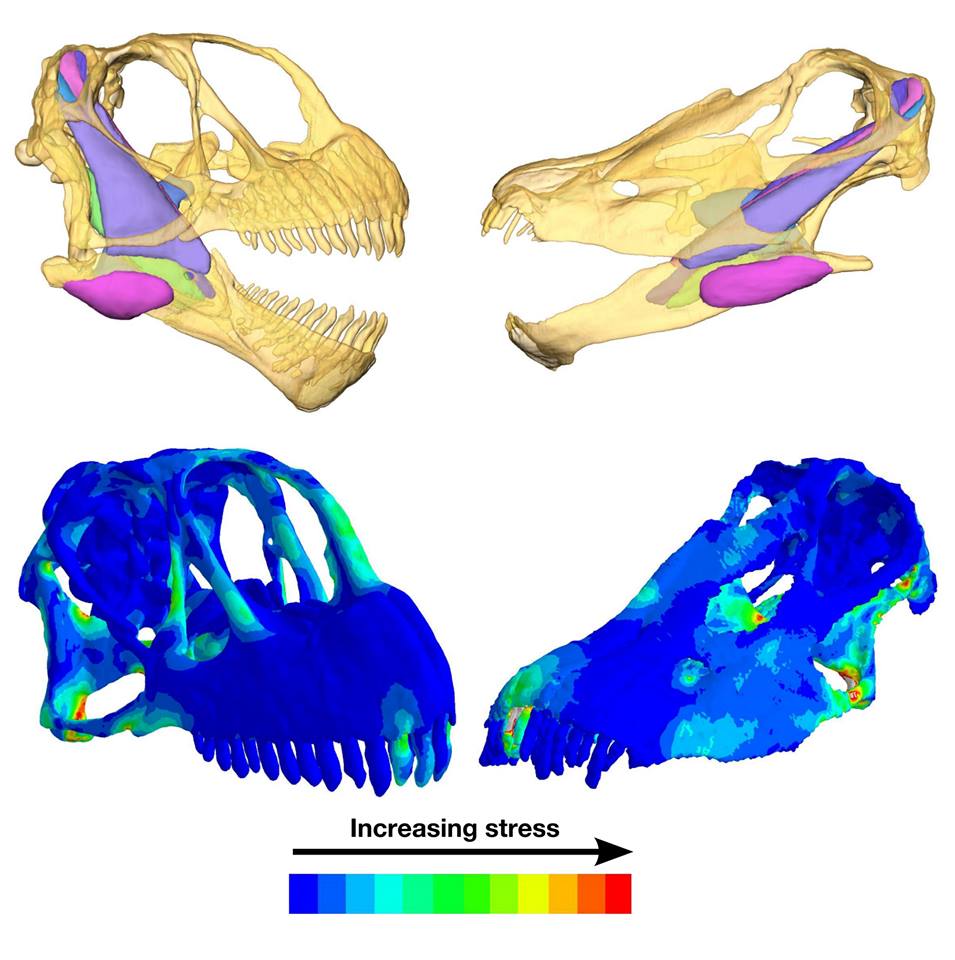
David compared the biomechanics of the skulls of Camarasaurus (left) and Diplodocus (right), as these taxa represent the end members of sauropod skull shape. The top images are virtual reconstruction of the skull, mandible and jaw musculature. The bottom images are finite-element analysis von Mises stress contour plots. Hotter colours refer to greater stress. Camarasaurus‘ skull has greater muscle mass and the teeth are angled more vertically than the skull of diplodocus. The stress plots show greater stresses in the biting teeth and palatal region of Diplodocus. Image credit: David Button. Modified from Button, David J., Emily J. Rayfield, and Paul M. Barrett. “Cranial biomechanics underpins high sauropod diversity in resource-poor environments.” Proceedings of the Royal Society of London B: Biological Sciences 281.1795 (2014): 20142114.
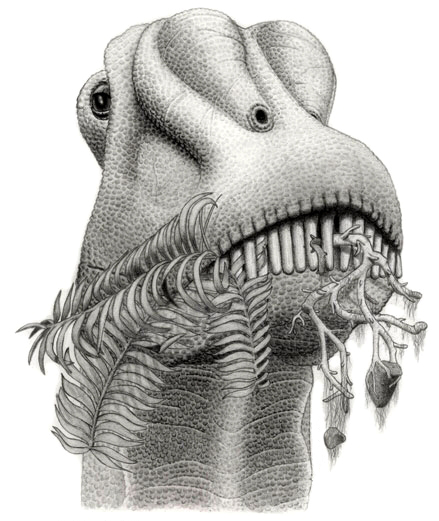
Finite element analysis also provided an opportunity to test various feeding behavior that had been suggested for sauropod dinosaurs. David has shown that Diplodocus skulls show less stress than Camarasaurus skulls during branch stripping – a mode of feeding in which teeth strip the leaves off a branch. Image credit: Jordan Mallon. Image from Wikimedia CC BY-SA 2.5.
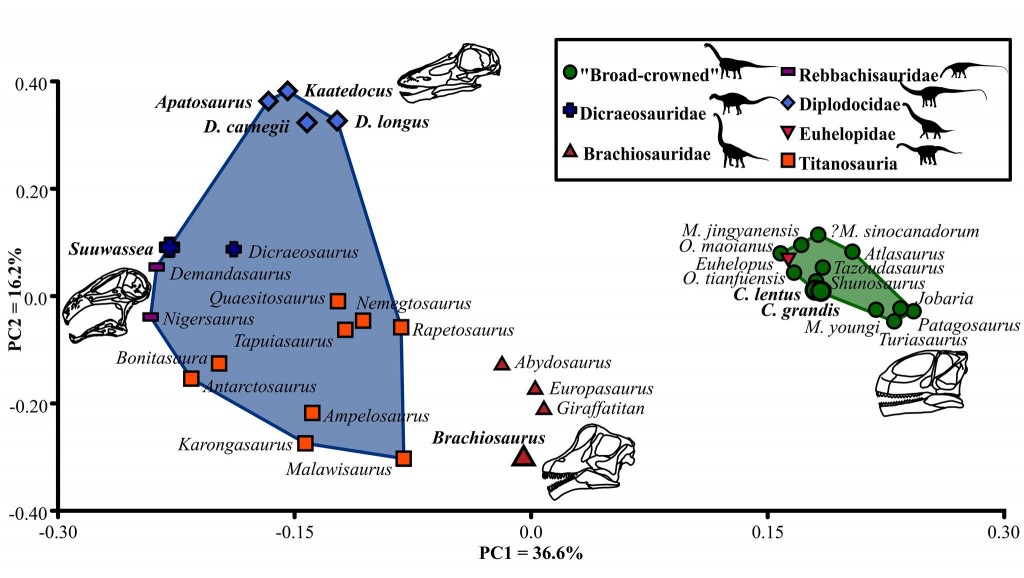
Using functional characters, and guided by the FEA analyses of Camarasaurus and Diplodocus, David could compare more broadly between the skulls of sauropods, including those from the Morrison. The image above shows a “functionspace” plot resulting from principal coordinate analysis of biomechanical and functional characters of the skull and teeth of sauropods. More positive values on PC axis one refer to greater bite forces. More negative PC2 values refer to more robust mandibles with occluding dentitions. More positive PC2 values indicate procumbent dentition and an elongated jaw joint, allowing sliding motions. Taxa from the Morrison Formation are indicated in bold: note that they are widely distributed in “functionspace”, indicating differences in feeding behaviour. This suggests that the sauropods of the Morrison Formation coexisted through ‘niche partitioning’ – the process by which species have evolved to use the environment differently, and thus avoid competing with each other. Image credit: David Button. Image modified from Button, David J., Emily J. Rayfield, and Paul M. Barrett. “Cranial biomechanics underpins high sauropod diversity in resource-poor environments.” Proceedings of the Royal Society of London B: Biological Sciences 281.1795 (2014): 20142114.